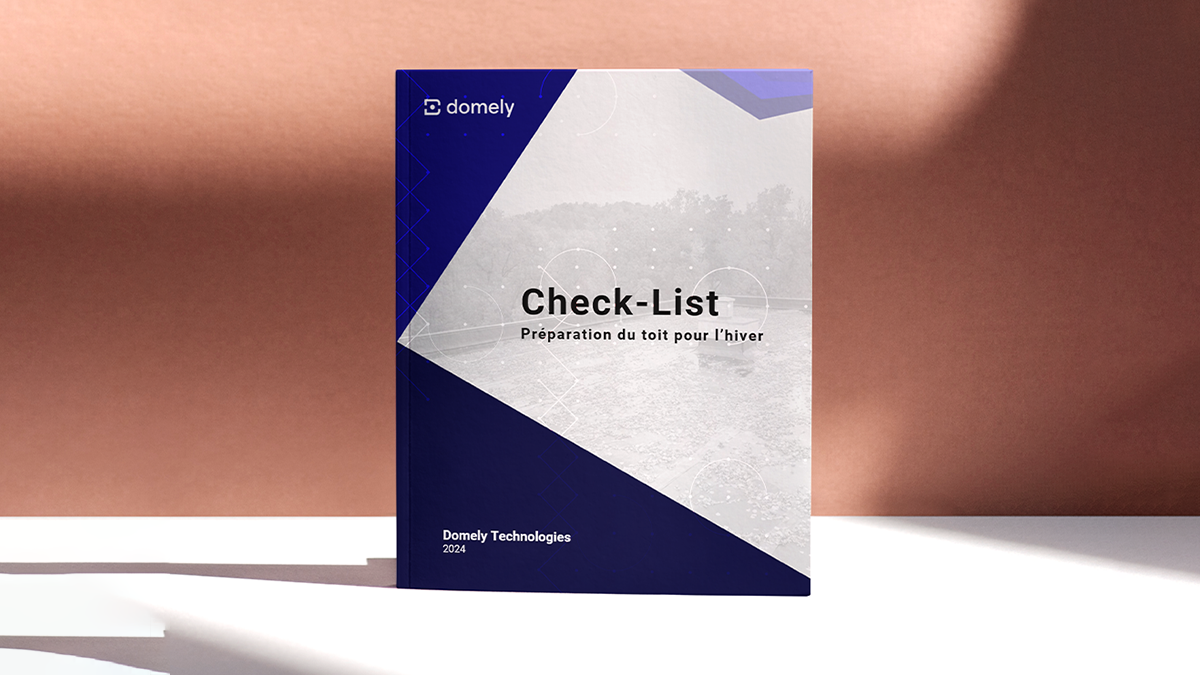
Climate Change is Changing the Rules - Why You Need a New Approach to Roof Snow Load
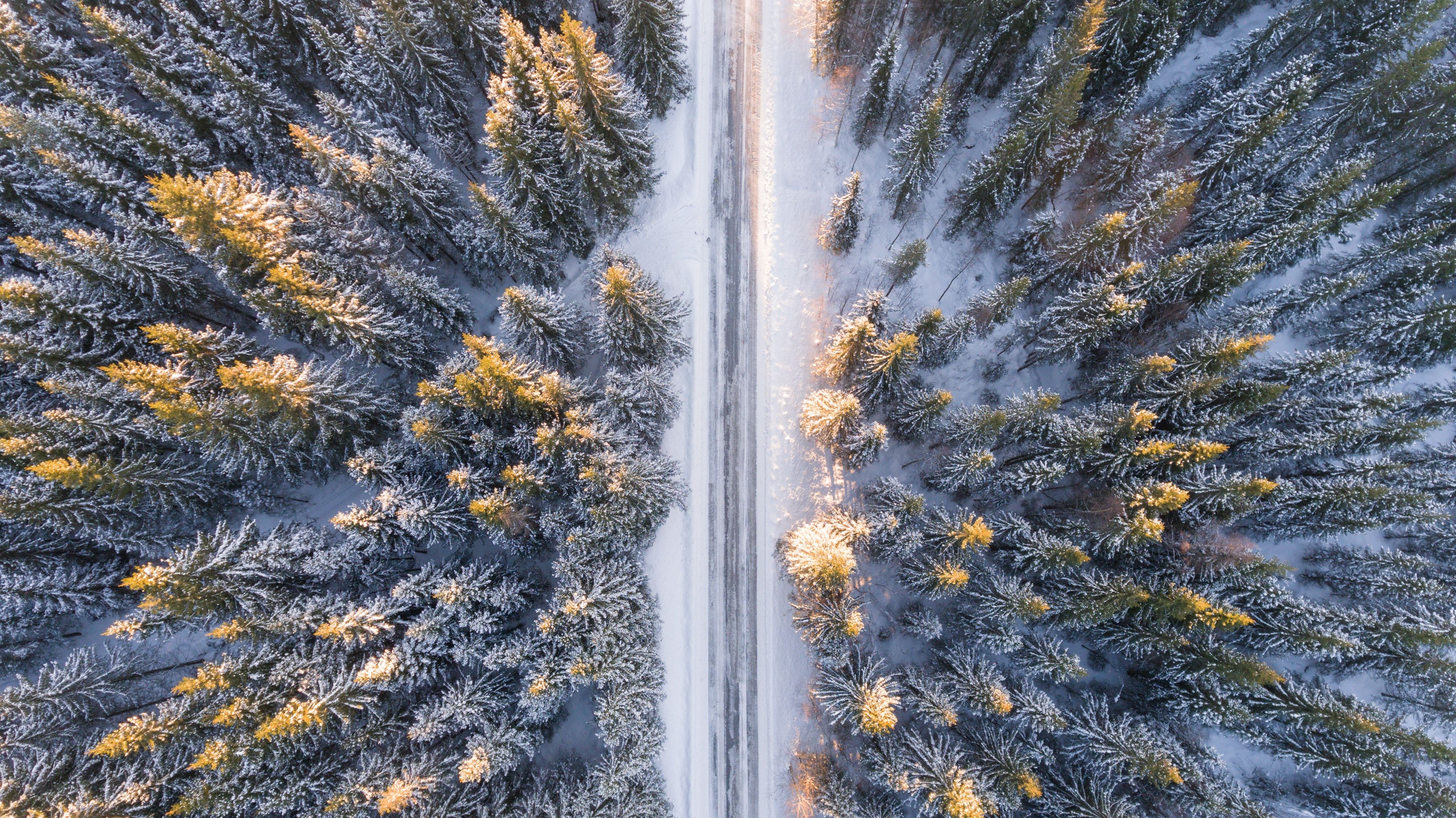
For decades, building codes and traditional roof snow load calculations have relied on historical weather data to ensure structural safety during winter. But climate change is rewriting the rules, making these outdated methods increasingly unreliable. With the rise of extreme weather events, unpredictable freeze-thaw cycles, and shifting precipitation patterns, a new approach to roof snow load is critical to safeguard buildings and ensure long-term resilience.
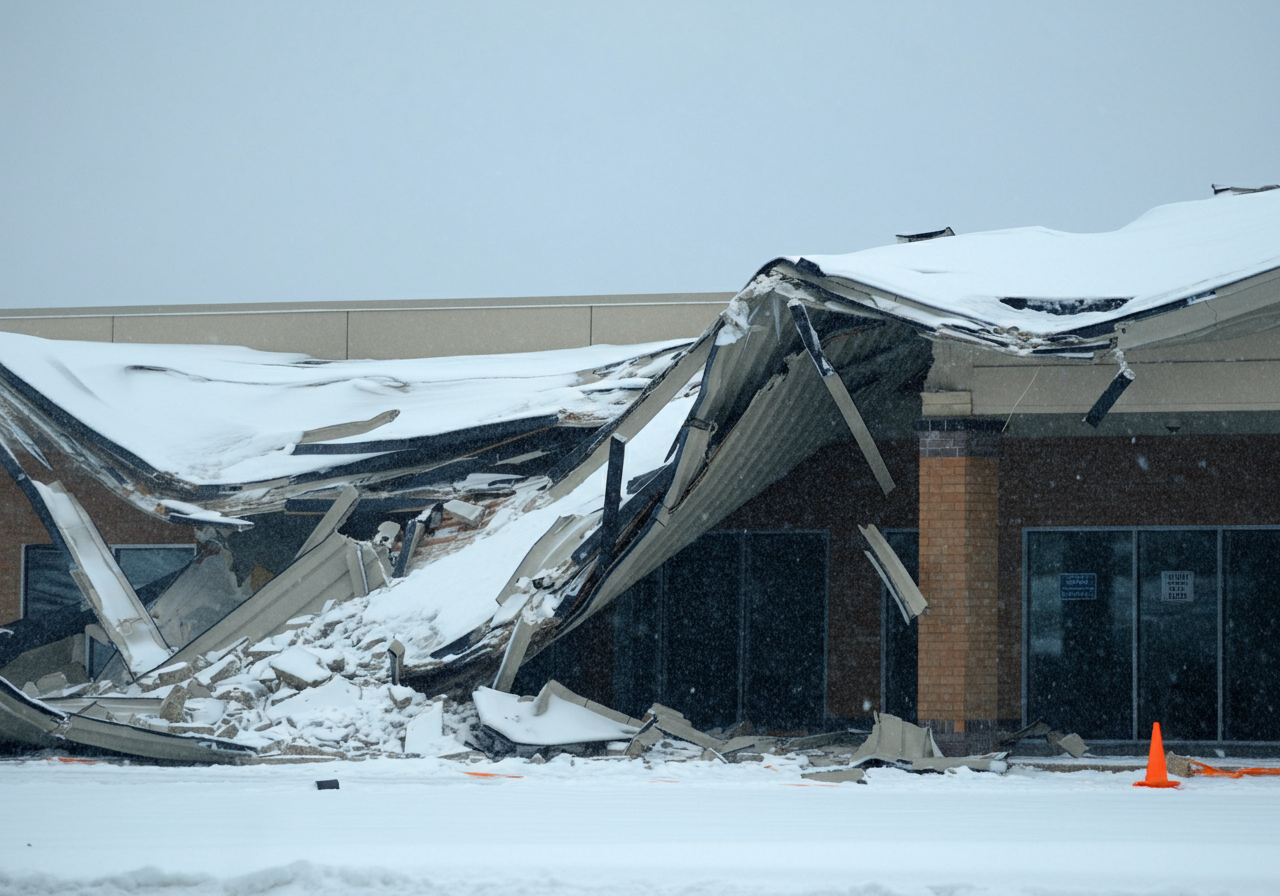
The Science is Clear: Climate Change is Here
The scientific community is unequivocal: climate change is a reality, and its impacts are already being felt around the world. The Intergovernmental Panel on Climate Change (IPCC), the leading international body for the assessment of climate change, has concluded that "it is extremely likely that human influence has been the dominant cause of the observed warming since the mid-20th century." (IPCC, 2013).
This warming trend is having a profound effect on weather patterns, including those related to snow.
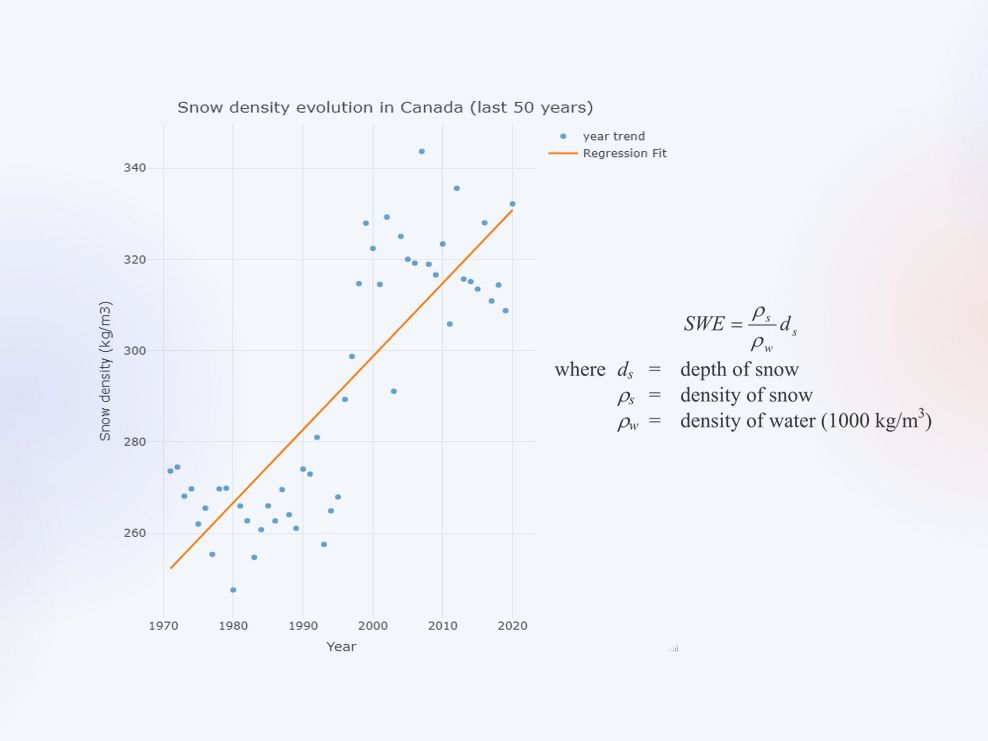
The Changing Nature of Snow
Climate change is altering the fundamental characteristics of snow, making it more challenging to predict and manage. These changes include:
- Increased Precipitation: In many regions, climate change is leading to increased precipitation, both in the form of rain and snow (IPCC, 2021). This means that roofs are being subjected to heavier loads than in the past. A study by the Ministère de l’Environnement et de la Lutte contre les changements climatiques (MELCC) in Quebec found that February 2019 had the highest water content in snow cover in the past 42 years (MELCC, 2019), thus rewriting what the new normal water content in snow is.
- Shifts in Snowfall Timing: Climate change is disrupting traditional snowfall patterns, with some areas experiencing earlier or later snowfalls, while others see more frequent and intense snowstorms (Kunkel et al., 2013). For example, a study by the U.S. Climate Change Science Program found that snowstorms have become increasingly more severe since the 1950s (U.S. Climate Change Science Program, 2008).
- Unpredictable Freeze-Thaw Cycles: Warming temperatures are leading to more frequent freeze-thaw cycles, which can cause snow to melt and refreeze, increasing its density and weight (Strasser, 2008). These cycles can create layers of ice within the snowpack, making it significantly heavier.
- Rain-on-Snow Events: The combination of rain and snow can significantly increase the weight of snow on a roof (Agence QMI, 2019). Rainwater can be absorbed by the snowpack, making it much heavier. Research has shown that this additional weight from high water content can be a critical factor in causing snow loads to surpass building capacity (Geis, Strobel, & Liel, 2012).
- Increased Snow Drifting: Changes in wind patterns can lead to increased snow drifting, creating uneven snow loads on roofs (Buckingham, Skalka, & Bongard, 2015). Research indicates that high wind speeds increase snow depth variability, creating uneven loads that can be difficult to predict (Winstral & Marks, 2014).
These changes are creating a new set of challenges for building owners, engineers, and roofing professionals.
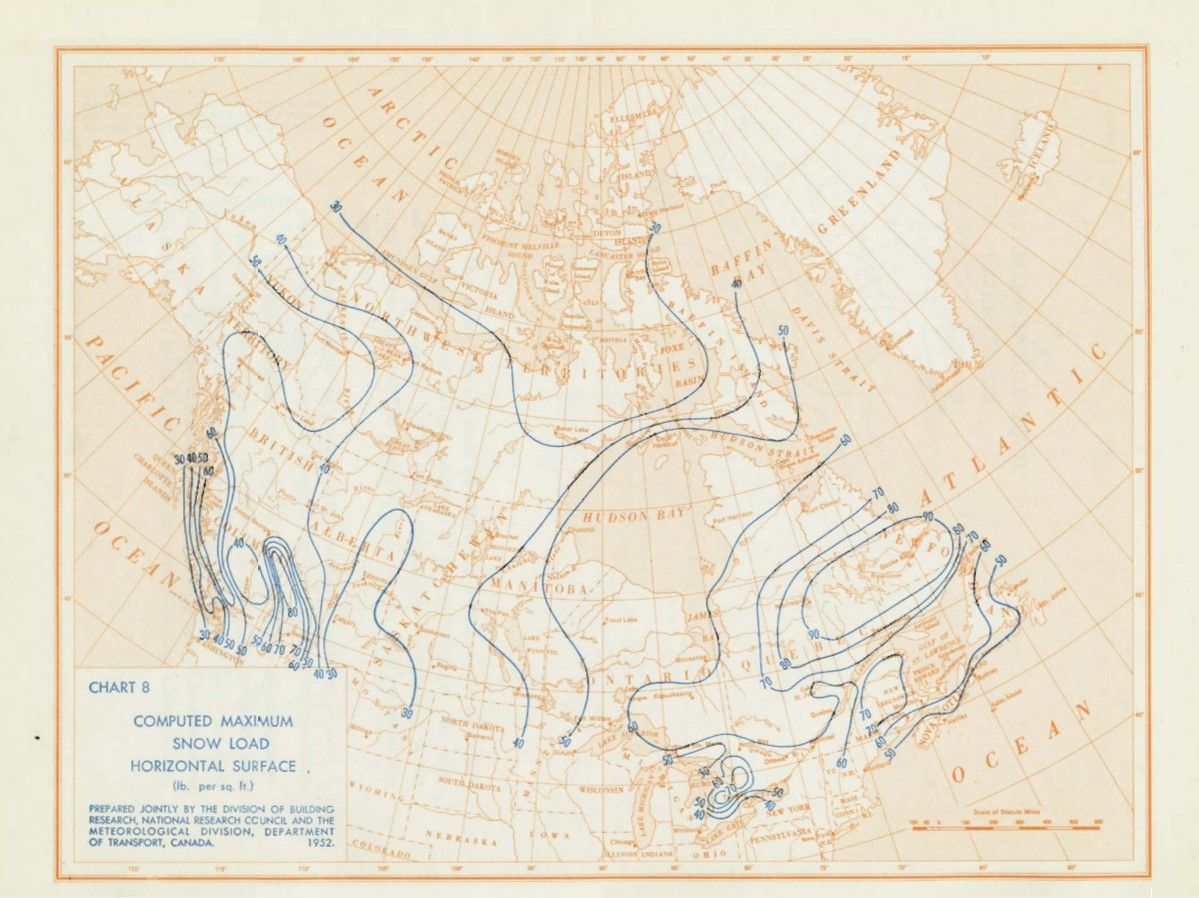
The Impact on Building Codes
Building codes are designed to ensure structural safety, but they are based on historical weather data, which is becoming increasingly irrelevant in a changing climate.
"The observed relationship between snow failures and snowfall is of particular interest given changes in global climate occurring worldwide," note researchers in a study on snow-induced building failures (Geis, Strobel, & Liel, 2012). They go on to warn that "Although the overall frequency of snowstorms is expected to decrease on a global scale, snowstorms have become increasingly more severe since the 1950s."
This means that building codes may no longer provide adequate protection against the risks associated with roof snow load.
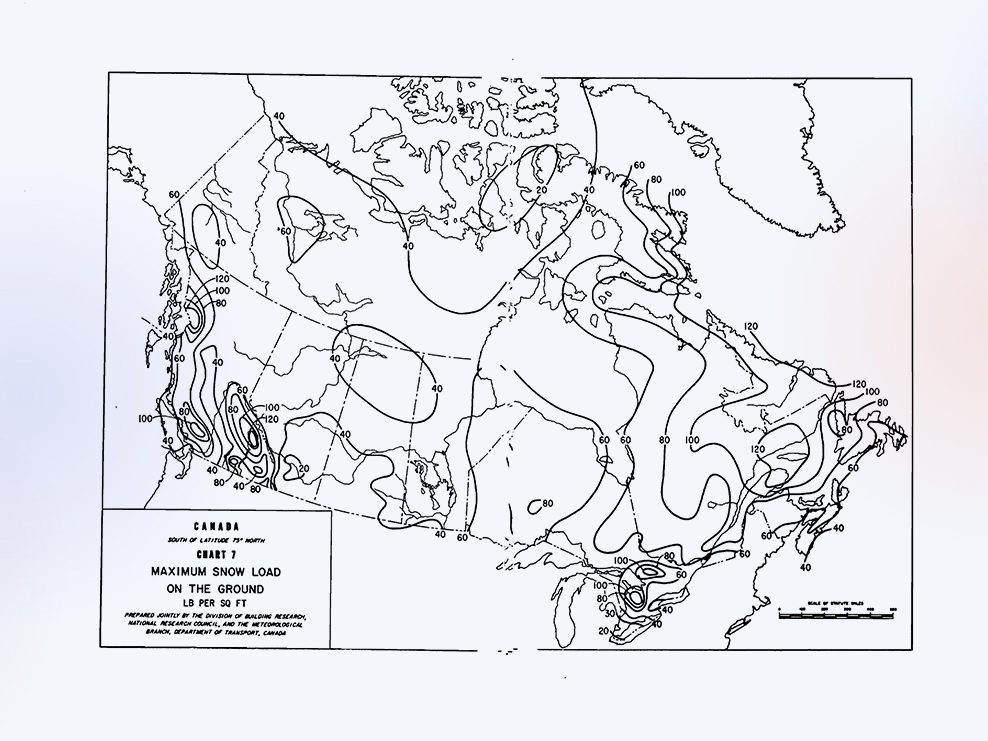
A Call for a New Approach
The changing nature of snow demands a new approach to roof snow load. We can no longer rely solely on historical data and static building codes. We need to incorporate real-time data, advanced technology, and a more dynamic approach to risk assessment. The call for a new approach is not new: the problem has been raised many times by the scientific community and governmental institutions. But the technology of the time made the task extremely complex and difficult to implement. Fortunately, recent technological advances have breathed new life into this hope of solving the problem once and for all.
Here are some key elements of a new approach:
- Real-Time Monitoring: Installing sensors on roofs to monitor snow load in real-time can provide critical data on the actual weight of snow and ice (Strasser, 2008). This data can be used to trigger alerts and inform decisions about snow removal . These sensors can measure factors like snow depth, density, and water content, providing a more accurate picture of the load on the roof.
- Advanced Predictive Modeling: By incorporating real-time weather data, advanced predictive models can provide more accurate forecasts of snow accumulation and weight (DeGaetano & Wilks, 1999). These models can factor in factors like temperature, wind speed, and precipitation type, allowing for more precise predictions of snow loads that exceed various return-period thresholds.
- Dynamic Building Codes: Building codes need to be more dynamic and adaptable to changing climate conditions (ALMahdi & al., 2020). This could involve incorporating real-time data and predictive modeling into code requirements. As climate change accelerates, it is crucial to upgrade hazard-related codes to ensure they reflect the annually changing nature of natural hazards (Molotch et al., 2005).
- Improved Drainage Systems: Ensuring proper roof drainage is essential to prevent water from pooling on roofs (Geis, Strobel, & Liel, 2012). This may involve upgrading existing drainage systems or installing new ones. Effective drainage systems can help mitigate the risks associated with rain-on-snow events and freeze-thaw cycles, preventing water buildup and potential structural damage.
- Enhanced Building Design: New buildings should be designed to better withstand the potential impacts of heavier snow loads (DeGaetano & Wilks, 1999). This could involve using stronger materials, increasing roof slopes, or incorporating features that minimize snow drifting. By considering the potential for heavier snowfalls in certain regions, new buildings can be designed with greater resilience against extreme weather events.
- Increased Awareness: Building owners, managers, and occupants need to be more aware of the risks associated with roof snow load in a changing climate (Strasser, 2008). This could involve providing educational materials and resources on snow removal and building maintenance. Educating stakeholders on the changing nature of snow and the increased risks can empower them to take proactive steps to mitigate these risks.
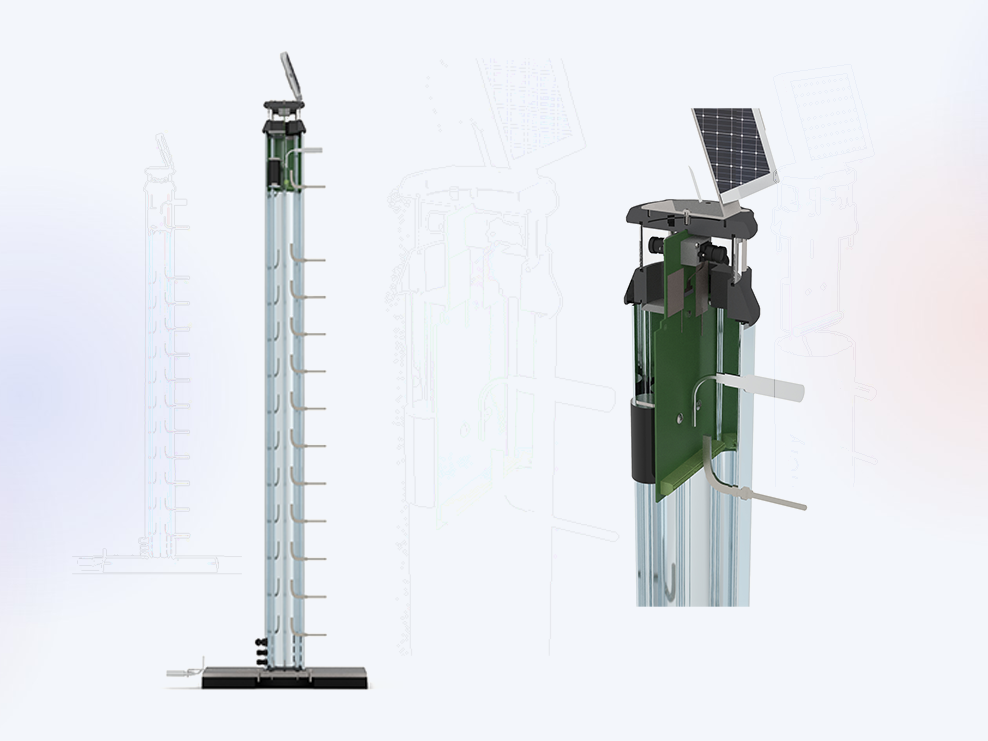
The Role of Technology
Technology is playing a crucial role in developing and implementing new approaches to roof snow load.
" Automatic roof monitoring stations consisting of a combination of meteorological sensors and a small snow pillow device is envisaged," suggests researcher Urs Strasser (Strasser, 2008), highlighting the potential of technology to "facilitate not only meteorological observations but also the monitoring and measurement of the actual snow load mass."
Companies like Domely are at the forefront of this technological revolution, developing innovative solutions that incorporate real-time monitoring, predictive modeling, and artificial intelligence to help building owners and managers proactively manage roof snow load.
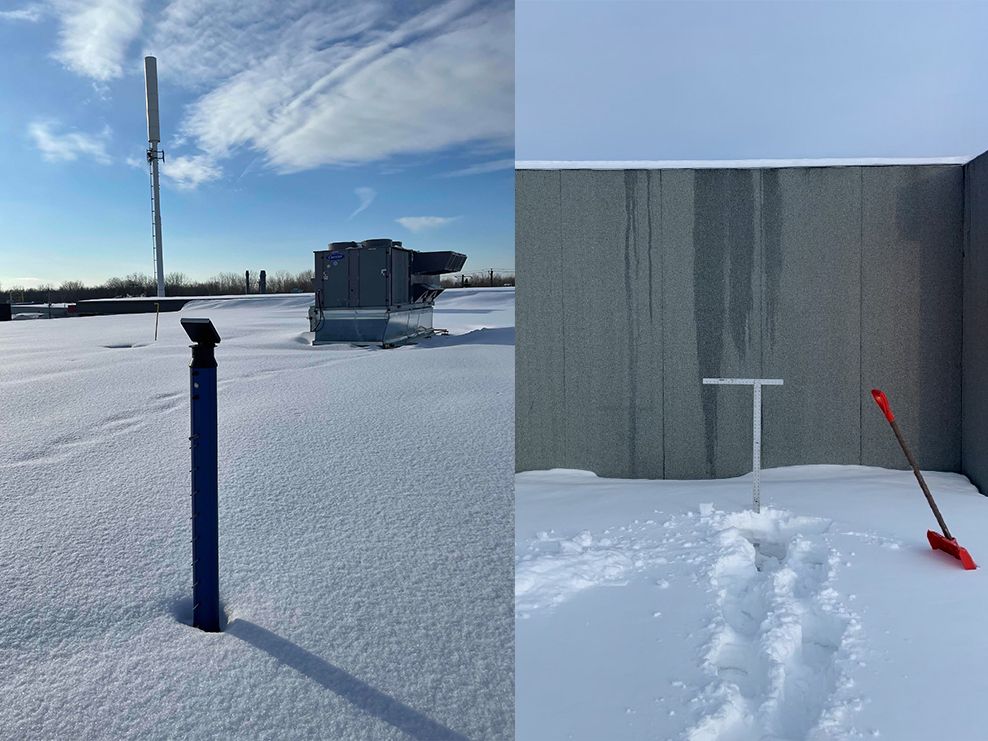
The Benefits of a Proactive Approach
A proactive approach to roof snow load can provide significant benefits, including:
- Increased Safety: By providing early warnings of potential overloads, proactive monitoring can help prevent roof collapses and other safety hazards.
- Reduced Costs: By optimizing snow removal efforts and preventing damage, a proactive approach can reduce long-term maintenance costs.
- Enhanced Building Longevity: By minimizing structural stress and preventing water damage, a proactive approach can extend the lifespan of a building.
- Improved Business Continuity: By preventing roof leaks and other disruptions, a proactive approach can ensure business continuity during winter.
A Critical Investment for the Future
In a changing climate, a new approach to roof snow load is not just a good idea, it's a critical investment in the future. By embracing real-time data, advanced technology, and a more dynamic approach to risk assessment, we can ensure that buildings are resilient and safe for years to come.
Don't wait for a disaster to strike. Take action now and implement a new approach to roof snow load . Your building, your business, and your peace of mind will thank you.
Sources:
- Agence QMI. (2019, February 18). Effondrement de toits enneigés: la ministre Laforest rappelle aux propriétaires leur responsabilité. Retrieved from Journal de Québec:
- ALMahdi, F., & al. (2020). Investigation of snow-induced collapse in bozüyük market. Engineering Failure Analysis, 118, 1-11.
- Buckingham, D., Skalka, C., & Bongard, J. (2015). Inductive machine learning for improved estimation of catchment-scale. Journal of Hydrology 524 , 311–325.
- DeGaetano, A., & Wilks, D. (1999). Mitigating snow-induced roof collapses using climate data and weather forecasts. Meteorological Applications, 6(4), 301-312.
- Geis, J., Strobel, K., & Liel, A. (2012). Snow-induced building failures. Journal of Performance of Constructed Facilities, 26(4), 377–388.
- IPCC. (2013). Climate Change 2013: The Physical Science Basis. Contribution of Working Group I to the Fifth Assessment Report of the Intergovernmental Panel on Climate Change. Cambridge University Press.
- IPCC. (2021). Climate Change 2021: The Physical Science Basis. Contribution of Working Group I to the Sixth Assessment Report of the Intergovernmental Panel on Climate Change. Cambridge University Press.
- Kunkel, K. E., Karl, T. R., Easterling, D. R., Redmond, K., Young, J., Andsager, K., ... & Huang, J. (2013). Probable maximum precipitation and climate change. Geophysical Research Letters, 40(7), 1402-1408.
- *MELCC. (2019, February). Faits saillants. Retrieved from Ministère de l'Environnement et Lutte contre les changements climatiques:
- Molotch, N., Colee, M., Bales, R., & Dozier, J. (2005). Estimating the spatial distribution of snow water equivalent in an alpine basin using binary regression tree models: the impact of digital elevation data and independent variable selection. Hydrological Processes, 1459-1479.
- Strasser, U. (2008). Snow loads in a changing climate: new risks? Natural Hazards and Earth System Sciences, 8(1), 1-8.
- Strobel, K., & Liel, A. B. (2013). Snow load damage to buildings: Physical and economic impacts. Proceedings of the Institution of Civil Engineers - Forensic Engineering 166(3), (pp. 116-133).
- U.S. Climate Change Science Program. (2008). Weather and climate extremes in a changing climate. Regions of focus: North America, Hawaii, Caribbean, and U.S. Paci?c Islands. A report by the U.S. Climate Change Science Program and the Subcommittee on Global Change Research. Washington, DC, USA.
- Winstral, A., & Marks, D. (2014). Long-term snow distribution observations in a mountain catchment: Assessing variability, time stability, and the representativeness of an index site. Water Resources Research, 50(1), 293-305.
Read more in this category
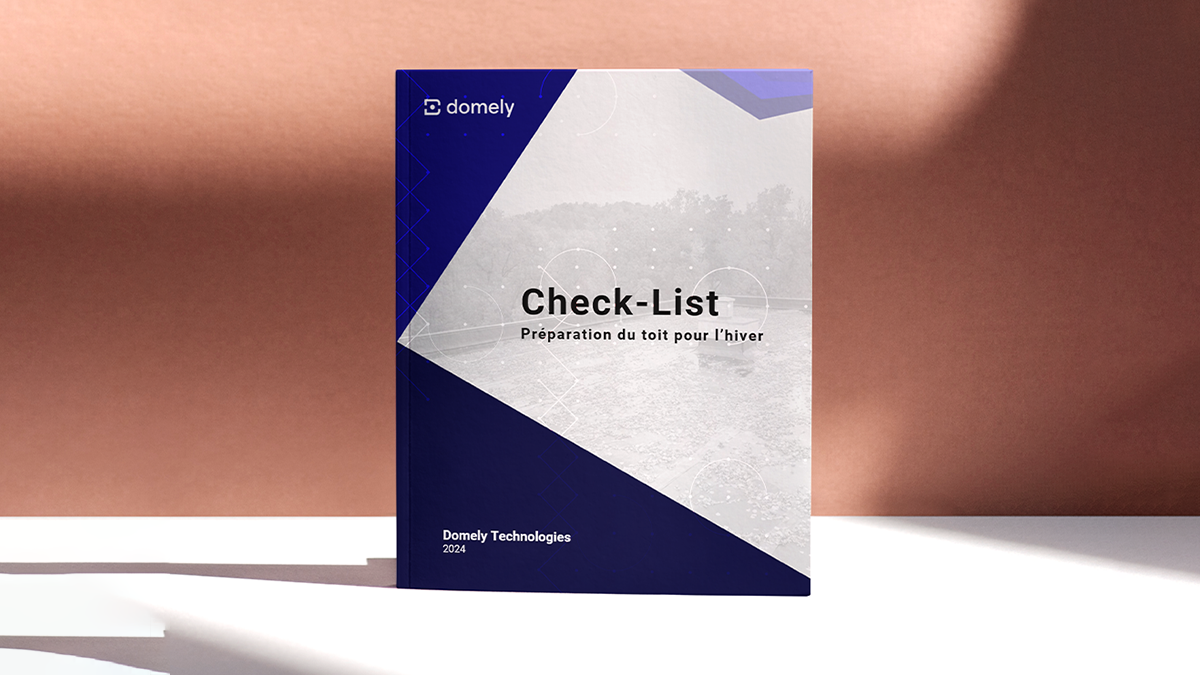
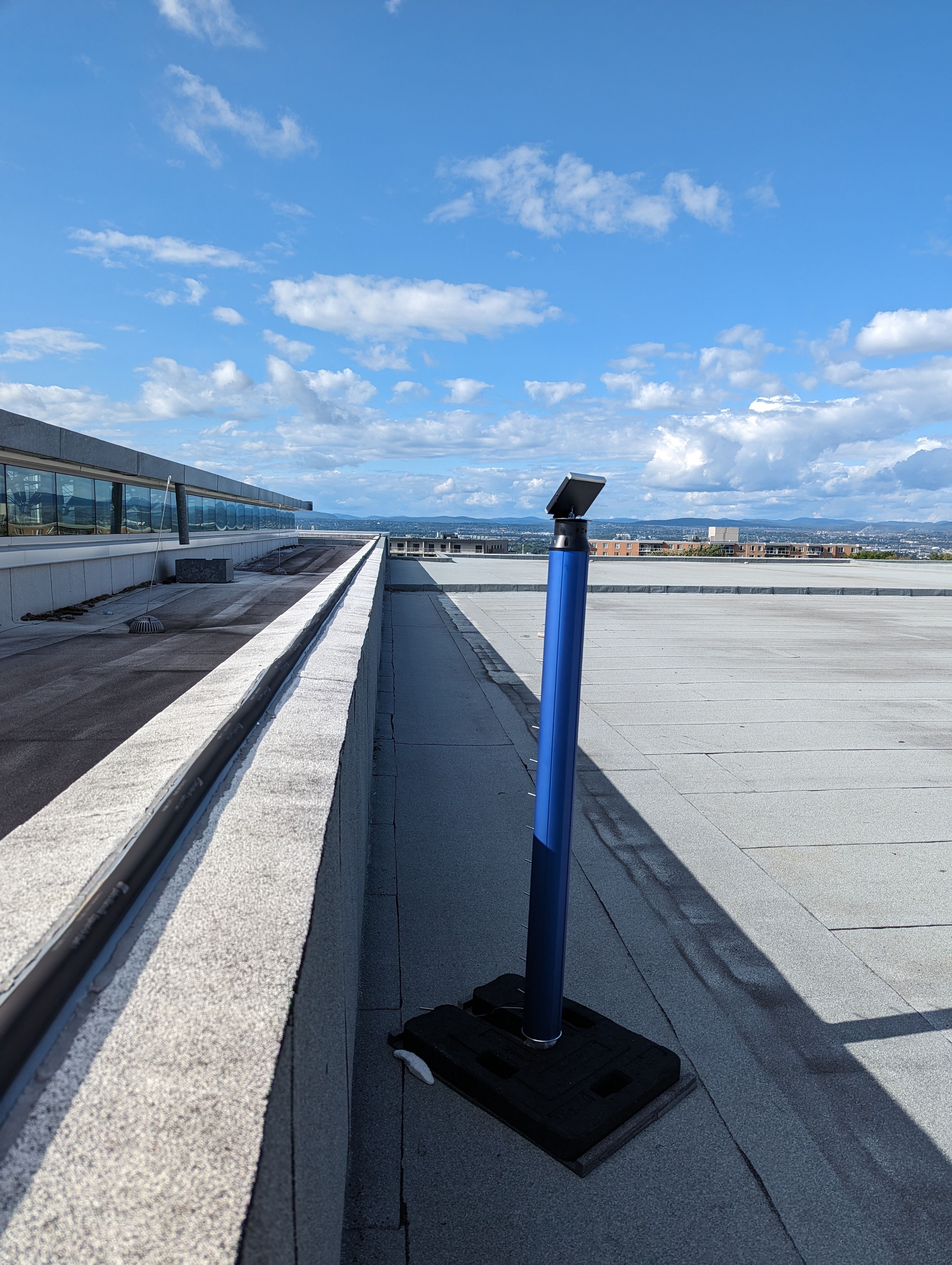
Case Study - Domely Connect™
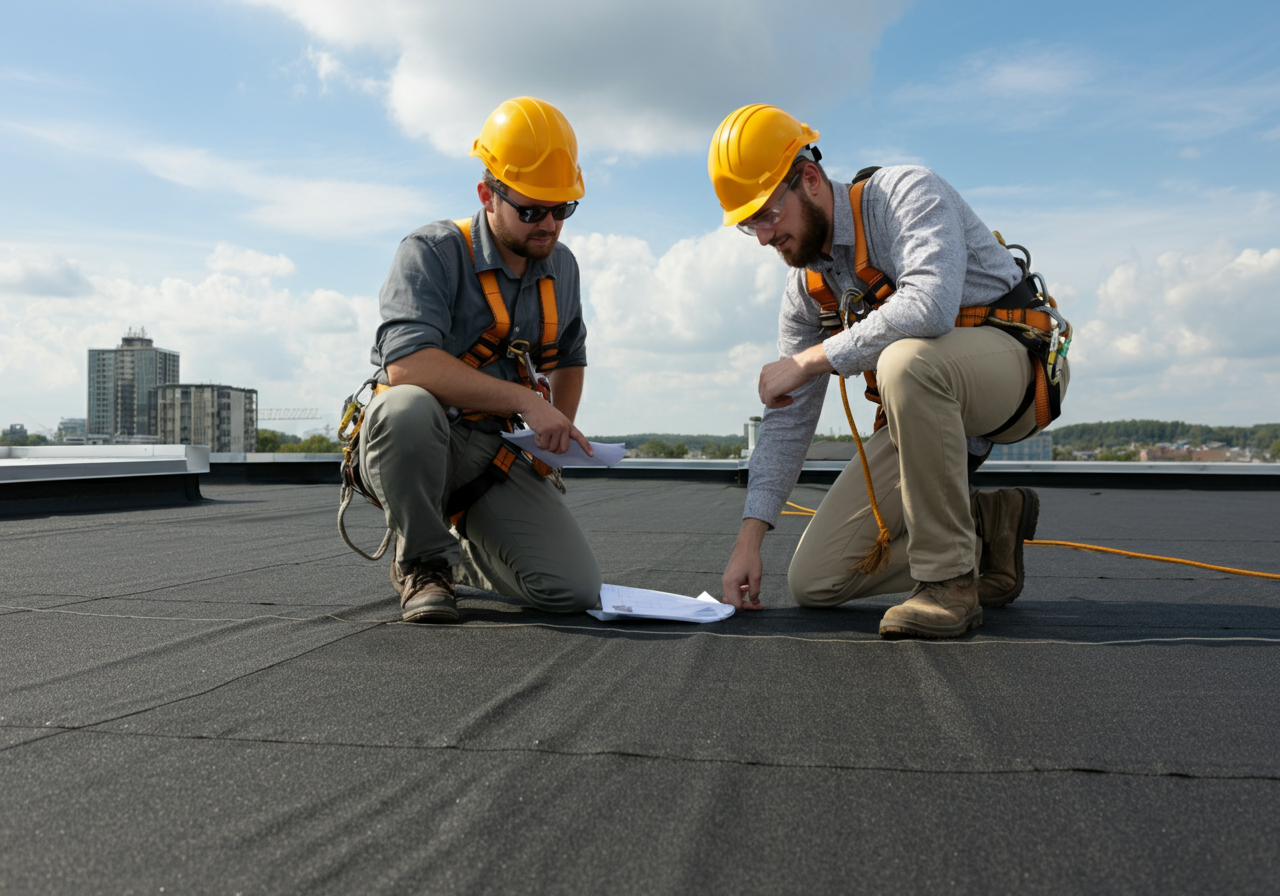
How to inspect your roof ?
Get a quote
Want a quote or have questions? Would you like to better understand how our smart offering and unique data can help you?